MYTHS
Myth:
We
put a man on the moon, didn't we? We ought to be able to grow papayas in
the Himalayas.
Reality:
We
cannot grow papayas in the Himalayas.
Fallacy:
Every
problem has a solution following it around like a dog on the end of a leash.
Reality:
There
are no solutions. Only subtler problems.
Fable:
A
simple cow-magnet attached to your carburetor will let your stationwagon
get over 100 miles per gallon.
Reality:
A
moped gets about 100 miles per gallon with or without cow-magnets. A stationwagon
won't.
Folklore:
The
oil companies are holding back. We can produce all the gasoline we'll ever
need.
Reality:
They
aren't and we can't.
Legend:
They
used to say that the human body was not capable of withstanding speed greater
than 40 miles per hour.
Reality:
"They"
were wrong.
Whimsy:
According
to some theory, the bumble bee can't fly.
Reality:
The
bumble bee can fly. What theory?
Fiction:
Nothing
is impossible
Reality:
That's
a quote from Peter Pan. Try striking a match on an ice cube.
Fantasy:
Some
day, nuclear power will be so cheap, they won't even bother to meter it.
Reality:
"They"
meter it.
Illusion:
Technology
in agriculture gave us the green revolution.
Reality:
Petroleum
in agriculture gave us the green revolution.
Chimera:
The
benefits of nuclear power far outweigh the risks.
Reality:
For
every foot of red carpet that nuclear technology lays before us, it takes
up a yard behind.
Myth:
Whenever
we've had a need in the past, some new technology always came along to
fill it.
Reality:
We
need a direct substitute for petroleum...
Electric Car
Not all policy-makers and not all citizens
appreciate the limits imposed by the laws of thermodynamics. Some folks
show blissful optimism.
"What's wrong with an electric car?" they ask.
Start with a particular number of gallons of
crude. Pump it out of the ground and ship it to the refinery. Crack it
down to heating oil. Transport it again, this time to the electric power
plant. The parcel of crude we started with has already shrunk quite a lot
in the refinery, and some was used up, indirectly, to supply the energy
for its own processing and transportation.
Next, burn up the refined oil to get heat to
boil water to make steam to spin a turbine to drive a generator to produce
electric power. There are heavy losses in all these steps, especially those
resulting from the thermodynamic inefficiencies of that turbine.
Now, put the electric power through a transformer
to produce high voltage. More losses. Distribute it along wires. More losses.
Transform it down to household level. More losses still.
Finally, rectify the current and charge the
bank of batteries in your little electric car of the future. There are
losses to account for here, too.
In the morning, unplug the thing and drive
off quietly to work. You have enough electric charge to get you there and
back again at moderate speed.
Consider for a moment what the outcome would
be if you refined the same amount of crude into gasoline and drove a conventional
compact car to work with it. You would have enough fuel to get there and
back again at moderate speed. The same distance, it turns out.
"Yes, but," policy-makers and citizens ask,
"can't the electric car be powered by nuclear-generated electricity?"
Yes, but...
Syn-Fuel
What we call progress is often marked by rediscovery.
Thus, the renewed interest in the lighter-than-air craft, the bicycle,
and the rediscovery of the versatility of coal as an energy resource.
Coal was the first of the fossil fuels to become
important. It is the world's most abundant fossil resource. There may be
as much as a 600 year supply on reserve. But coal is unsuitable as a direct
replacement for petroleum, particularly for internal combustion engines.
Long ago, it was learned that clean fuels of
many types can be made from coal. "Coal oil" is still the common name used
in the rural U.S. for kerosene. It was produced from coal in the mid-19th
century. "Town gas" or "water gas" has been produced from coal for use
in urban lighting and cooking for a period of time extending well into
the 20th century.
During WWII, Germany demonstrated the technical
feasibility of a large-scale coal conversion technology. Under price controls,
cheap oil and gas returned to the marketplace after WWII, which led again
to a general decline in the importance of "King Coal."
Awareness that the petroleum age is coming
to an end provides new impetus to develop a technology for producing low
cost gases and liquid fuels from coal. Here we find that public expectations
may be somewhat unrealistic.
Synthetic fuel from coal is unfortunately distinguished
from other rediscoveries. The technology is not economically superior to
its predecessor, petroleum. Based on intrinsic costs, synthetic fuels are
50 times more expensive than crude oil.
The requisite capital investment in technology
development, plant, and equipment for coal processing is measured in billions
of dollars. The only basis for revenue parity, though, is predatory pricing
by a cartel. With the mere stroke of a pen, OPEC has been known to reduce
prices, unthinkable as that once was. How then will the massive investments
ever be repaid'?
Would you like to sell your bike to buy some
stock?
Water Gas
In the post-petroleum age, we'll have to find
a way to run vehicles on water. Here's how.
Coal is almost entirely carbon. Synthetic fuels
must be hydrocarbons, molecules made of hydrogen and carbon. The basic
requirement, therefore, is to add hydrogen to the carbon atoms in coal.
In addition, heat must be supplied to the process.
This heat subtracts from the total energy released by the synthetic fuels.
In general, the reliance on coal-based "syn-fuels" will lower the efficiency
with which we use our primary resources by a factor of three. Keep that
in mind.
Hydrogen is usually provided by reacting coal
with steam. Water vapor. The most serious problem faced by synthetic fuels
plants is their need for water. Not only is water one of the raw materials,
but it is also needed for cooling. It takes two tons of water for each
ton of coal processed, about twice the needs of an electric power plant
of the same energy output.
By the way, only open-pit coal is economical
enough for synthetic fuels processing. In the U.S. the most promising plant
sites are either in the arid Southwest, in the Four Comers area, or in
the equally arid North Central region of the Dakotas, Montana, and Wyoming,
where agriculture and industry compete for water.
Bicyclists need water, too. Not very much,
though.
Yellowcake
Just before noon one day in late 1973, a certain
bicyclist walked uninvited into the office of a distinguished econometrician
and sat down.
"What do you think of yellowcake?" asked the
bicyclist.
"It is nearly time for a luncheon appointment,"
replied the man of numbers. "You are not invited. Happy birthday, just
the same."
"Yellowcake has nothing to do with birthdays."
"Of course not," the economist-turned-statistician
said. He tapped his pipe into an ash tray on the desk beside his computer
terminal. "Yellowcake is uranium ore, am I correct?"
The bicyclist nodded. "When was the last time
you analyzed what is happening with yellowcake?"
The gifted econometrician took off his reading
glasses and shrugged. He ran his fingers through his graying forelocks
and smiled patiently at the bicyclist. "When was the last time you drove
a car?"
"But you do know about uranium pricing."
"You'll have to excuse me, but I do have to
get a move on or I'll be late. Maybe we can talk about the price of yellowcake
later."
"The price has been going up sharply the past
few months," declared the bicyclist.
"The prices of many things have gone up lately,"
said the man who had been deservedly acclaimed for predicting as much.
"It's because of the energy crisis," said the
bicyclist.
"Good work, oh man of the wheel," said the
econometrician, always generous with his praise. "And do you remember the
rest of what you've been taught about supply and demand?"
"This may be a little different."
"Different?" The expert's analytic eyes sparkled
with conviction. "With petroleum shortages worldwide, one might expect
the price of uranium ore to go up. It is an alternative energy source,
is it not?" The econometrician stood up and strode confidently to his private
coat closet, one of the perquisites of his station.
"What's different," said the bicyclist, "is
that the costs for enrichment vary inversely with the quality of the ore
mined, not just with increased demand. The quality of uranium ore has been
steadily getting worse and the processing costs have gone from something
like $5.00 per pound to nearly $7.00 per pound.
The econometrician returned from the closet
with his arm in one sleeve of his overcoat. The bicyclist stood respectfully
and assisted him with the other sleeve. "Thank you," said the older, more
knowledgeable man. "The unit price of petroleum has gone up by those kinds
of percentages, and uranium enrichment is a petroleum-intensive process.
Again, what differentiates the cost increases for yellowcake from... any
other 'cake'?"
"Along with the unit price increases, there
are also more units of petroleum being consumed in the refining process
due to the lower quality of yellowcake now being mined."
"Look, I don't really have time to give you
a long lesson today. Suffice it to say, when the yellowcake gets to some
level, $10.00 per pound, say, the cost of nuclear-generated electric power
will reach an uneconomic level, and the unseen economic hand will cause
a shift away from nuclear power until the operating costs of fossil-fueled
generating plants catch up. Given the conditions in the Middle East, that
won't be long either."
"You're undoubtedly right, but there's just
this one other thing."
"Better make it fast.”
"Ok," said the bicyclist. "When you consider
the energy content of the enriched uranium, not just the dollars -- "
"Energy content?" asked the econometrician.
"Yes, for grades of yellowcake requiring as
much as $12.00 per pound of processing costs, the energy consumed to do
the enrichment exceeds the energy eventually obtainable from the uranium!"
The older man looked at his watch and grimaced.
"I must leave at once. You go ahead and think about these problems. I have
no doubt that you'll figure out how the law of supply and demand will prevail
and bring the world's mix of energy sources into equilibrium. Life is full
of economic trade-offs." And, with a wave of his hand, the econometrician
dashed off down the corridor, calling back over his shoulder. "Goodbye
for now!"
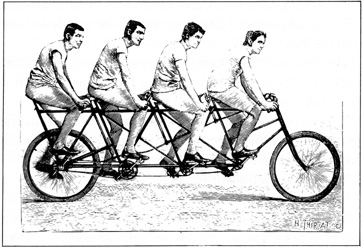
That same afternoon, the bicyclist was surprised
to look up from his own desk and to see the venerable econometrician bounding
through the doorway in shirtsleeves, eyes animated by some intense challenge
to his powerful mind.
"There is something different going on here,"
he exclaimed.
"With the yellowcake?" asked the bicyclist.
"Yes. of course. This business about energy
content has me stumped. It is energy yield we have to be concerned about
from here on. But that's physics, not economics."
"As you suggested” said the bicyclist, "I have
been thinking. In physics, even a small difference in degree can produce
a difference in kind."
"Can you be more concrete?" asked the econometrician.
The bicyclist cleared his throat and groped
for the precise words.
"A body," he said, "with a specific gravity
of 0.99 differs in density from a body with a specific gravity of 1.01
by a very small amount."
"By only two percent," said the econometrician.
"So?"
"One of them floats."
Breeder
The worst menace to our cities has to be the
LMFBR, an unconventional nuclear power plant with an awkward name, Liquid
Metal Fast Breeder Reactor.
Uranium comes in two forms, isotopes. One is
useful, the other is unuseful but abundant. Actually, just less scarce.
Even the useful uranium must be enriched before it can be used as fuel
inside a nuclear reactor. There, through fission, uranium makes heat, then
through a turbine-driven generator, electricity.
The useful uranium, of course, gets used up.
Yet, nuclear fission should not be confused with burning, although the
machine that uses up the uranium is often called a burner reactor, probably
to distinguish it from the breeder reactor.
You've doubtless heard some good things about
the breeder reactor. "It will produce more fuel than it uses," enthusiasts
proclaim. Seems almost like a miracle, a free thermodynamic lunch. Bicyclists
and others have learned to be skeptical of such Utopian rhapsodies.
The breeder reactor does use useful uranium
for fuel just as the burner reactor does, except that the breeder also
takes some of the unuseful uranium and breeds it into a useful fuel. Plutonium.
You've doubtless heard some bad things about
plutonium. "It's so radioactive, only a few pounds could kill every living
thing on earth," detractors proclaim. "Plutonium doesn't decay for hundreds
of thousands of years," they cry. "And you can make atomic bombs out of
the stuff, too!"
Nevertheless, the breeder reactor is regarded
by many policy-makers as a promising energy alternative precisely because
it does produce plutonium. Later on, some of the plutonium gets used up
in the same reactor to make heat, then electricity.
It takes quite a long time, decades, in fact,
for a breeder reactor to breed enough plutonium for its own use. Even a
fast breeder. All the while, the breeder acts like a conventional burner,
using up useful uranium. Since there's about ninety times as much unuseful
uranium in nature as there is useful uranium, the wait seems worthwhile.
But, if we ever intend to use breeders, we'd better hurry.
We don't want to burn up all the useful uranium
as nuclear kindling before we can light up the plutonium "fires" in breeders.
Enthusiasts are being somewhat generous, therefore,
in representing the breeder reactor as actually producing more fuel than
it uses. It merely salvages some of the unuseful uranium tailings.
You're probably wondering about the LMFBR.
Dystopia! The thing must eventually be abandoned. But not just because
of plutonium or some worrisome nuclear incident. There is also an old-fashioned
chemical problem in the LMFBR.
Circulating deep within all nuclear reactors
is a fluid called a coolant. Don't be misled by the term. It's anything
but cool in there. And, unless you've been living in a cave, you already
know what might happen if a nuclear reactor loses its coolant.
A burner reactor like Three Mile Island uses
water as a coolant. A fast breeder reactor uses a liquid metal, the
LM in LMFBR. In particular, sodium.
It's hard to find a more chemically reactive
element than sodium. A speck of it, dropped in a beaker of water, causes
a minor explosion. Except in a chemical lab or a fire-works factory, you've
probably never even seen pure sodium. Yet, for technical reasons, liquid
sodium is the coolant of choice in the LMFBR. Moreover, unlike water, the
stuff freezes at high temperatures, clogging pipes and hopelessly jamming
pumps.
Perhaps you can imagine how perilous future
LMFBR projects will be for our cities.
Lithium Six
In the post-petroleum age, some people expect
fusion reactors to produce gigantic amounts of "safe" heat.
It'll probably be safe enough. The problem
seems to be getting the things going, not shutting them off. Fusion reactors
should have no propensity for "melt-down," and they'll produce no radioactive
wastes.
The high temperatures in a fusion reactor,
tens of millions of degrees, make possible the most thermodynamically efficient
heat engines ever built.
And talk about recycling! Using the waste heat
from a fusion reactor, you can reduce any kind of trash to a plasma of
pure chemical elements.
Fusion reactors are like pieces of the sun
installed on the surface of the earth. Accordingly, some experts say they'll
raise the temperature of the biosphere. The main concern isn't that, however.
It's fuel.
The sun uses plain hydrogen for fuel. That
won't work on earth for technical reasons having to do with our low gravity
field. Fusion reactors here must use both heavy isotopes of hydrogen, deuterium
and tritium.
Deuterium is abundant in nature. One water
molecule out of 5,000 has a deuterium atom in it. Enough in the oceans
for countless millenia, except that for each deuterium atom, the fusion
reactor must have one tritium atom. Tritium is radioactive, with a half-life
of about twelve years, and thus is all but absent in nature.
Tritium is best produced by breeding a particular
metallic isotope; lithium six. The next question is, how much lithium six
is there?
Some estimates put it this way: If all the
known lithium six were bred into tritium and consumed in fusion reactors,
the amount of energy produced would correspond to that of our total worldwide
petroleum reserves.
The "fusion age," then, might last about as
long as the petroleum age. Not indefinitely. The bicycle age wouldn't begin
for another century or so.
But first a fusion reactor has to be made to
work.
Fusion
Fusion might be the ultimate energy technology.
Certainly the mightiest. Conceptually, fusion has many advantages over
fission. Just what we'll need in the post-petroleum age.
Fusion happens when atomic nuclei are held
together with sufficient force to cause them to, well, fuse. It produces
unimaginable amounts of energy.
Thus shines the sun from ninety-three million
miles away. Here on earth... the hydrogen bomb.
To harness this ferocious energy source for
peaceful uses, technologists must find a way to sustain controlled thermo-nuclear
reactions. High operating temperatures make containment a seemingly intractable
problem. At 50 million degrees, all known materials vaporize. Out of what
do you build a reaction chamber?
One of the leading ideas is called a "magnetic
bottle." Another concept uses giant lasers. Both present horrendous technical
difficulties.
Despite hundreds of millions spent on research
worldwide, even the initial goal of achieving a positive-yield fusion reaction
continues to slip further into the future, often by as much as a decade
per year.
At that rate, by the end of this century, success will be projected
beyond the end of the next.
Not much for wishful thinkers to hang their
hopes on. |