 Solvers
of
the World's Fastest Train
puzzle will recognize the photograph on the right as
that of the V150,
the Alstom
train that set a world record speed of 574.8 kph
(357.0 mph) on April 3, 2007, as recorded in this video.
That
the view is from the rear of the train can be confirmed
by the red lights and by the unoccupied cab. There is a
third clue: The pantograph
has been deployed. Whereas the V150 has a power
car at each end of the consist,
each with its own pantograph, the pantograph only at the
rear of the train is used to collect power from the overhead
system for distribution throughout the train.
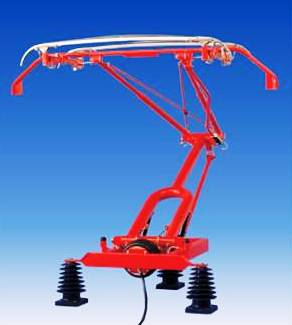 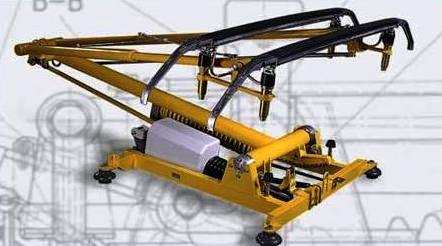
Pictured above are two examples of
modern pantograph design:
the Schunk
Model WBL
on the left and the Faiveley
Model EPDE
on the right. Both are designed for high speed
trains, featuring integrated pneumatic controls,
individual suspension of carbon collection strips,
vertical head guidance and fast lowering
devices.
For collecting electrical
power, the sleekest trains in the world,
traveling at hundreds of miles per hour,
continue to deploy awkward contraptions on
their roofs, which have the aerodynamic
properties of a child's backyard swing-set. |
Most
people
will recognize these devices, for they apply the same pantograph design as those
simple spring-loaded assemblies atop modern light
rail trains placidly plying their streetcar
services on trackways embedded in the middle of city
boulevards.
Unchanged in concept for decades, the
standard configuration is shown in Sketch 1 on the
right, with its main components called out:
stabilizing arm that keeps the pantograph head level
and control arm that actively moves the head
vertically to keep the force on the contact wire
within a narrow range. In high-speed trains, the
practice is to lock the lower arm in a fixed position,
which reduces the inertial mass that has to be raised
and lowered in real-time.
Overcoming wind resistance of the pantograph
would seem to be a minor challenge for any train
developing thousands of horsepower. Still, the
V150 deploys only the rear pantograph on each consist
and has roof fairings over the idle pantograph stowed
in front.
Meanwhile, automatic controls in the
pantograph system must maintain the requisite contact
force, adjusting rapidly for variations in the track,
overhead wire, and swaying of the train as indicated
in Sketch 2.
Finally, under emergency conditions,
the control system must be designed to drop the
pantograph abruptly. Watch this clip
for an example of what can go wrong.
The
Pantograph Design puzzle
will address another issue -- aerodynamics -- which is
receiving plenty of attention in the high-speed rail
industry. Let us first take notice of the fact
that modern Z-shaped
pantographs are not symmetrical with respect to
direction of train travel: Going one way, the
"knuckle" between the pantograph arms will be facing
forward, which means the carbon strips are being drawn
along the wire. In the opposite direction, the
"knuckle" will be facing backward and the carbon
strips will be pushed along the contact
wire. For light-rail vehicles operating no
faster than, say, 50 mph, there are no significant
aerodynamic effects resulting from the train's
direction. At speeds over 100 mph, though, one
direction must surely be preferable.
Have a look at the picture of
the Acela
below, which is a view from the front of the train,
and we can see the "knuckle" is facing backward, so
that the contact strips are being pushed along
the contact wire. The picture on the right shows
a close-up of the pantograph. It shows something
else, too...
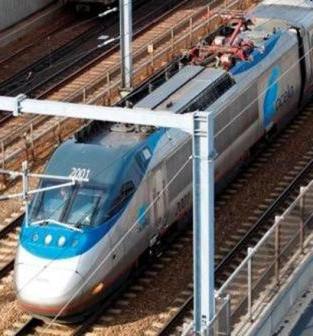
On Acela trains, each power
car is equipped with two pantographs.
They face in opposite directions. Unlike the
V150, which distributes traction power to all cars in
the consist from the rear pantograph, the Acela
applies traction power only in the power car itself
collected from overhead by one of its own pantographs
-- always the pantograph selected for its "knuckle"
facing the rear of the train.
The
rationale
for the rear-facing "knuckle" will become clear to
solvers of the Pantograph Design
puzzle using a hypothetical train. Let the upper
arm on its pantograph be six feet in length. When
deployed at a nominal level, the pantograph reaches
upward from the 'knuckle" a distance of three feet to
the contact wire.
The frontal areas of the contact strips and
their holders are held perpendicular to the relative
wind and dominate the aerodynamic drag
of the structure. As shown in Sketch 3, with the
"knuckle" facing aft, drag imposes an upward component
of force ("lifting force") on the head. Solvers
will observe that traveling in the opposite direction,
the force will be pointing downward.
What are your estimates for the
following table entries:
Cruise
Speed
|
Force Against
Contact Wire
|
Aerodynamic
Drag
|
Lifting
Force
|
mph
|
lbs
|
lbs
|
lbs
|
0
|
40
|
0
|
0
|
50
|
40
|
16
|
?
|
150
|
40
|
?
|
?
|
250
|
40
|
?
|
?
|
350
|
40
|
?
|
?
|
GO TO SOLUTION
PAGE
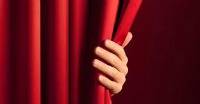
|